Time-resolved Hall effect measurements
The Hall effect is one of the oldest and most useful effects for the characterization of the electric and magnetic properties of metals and semiconductors. The ordinary, anomalous, planar, and topological Hall voltages have become standard tools for studying the physics of metallic, semiconducting, insulating, and topological materials, as well as for assessing the performance of electronic and spintronic devices. Unfortunately, their use has been so far restricted to dc or low-frequency ac applications by the inherent small magnitude of the Hall signals, which hinders their time-resolved detection. This limitation represents a serious obstacle to study the dynamics of magnetic and electronic systems, both in the classical and quantum regimes.
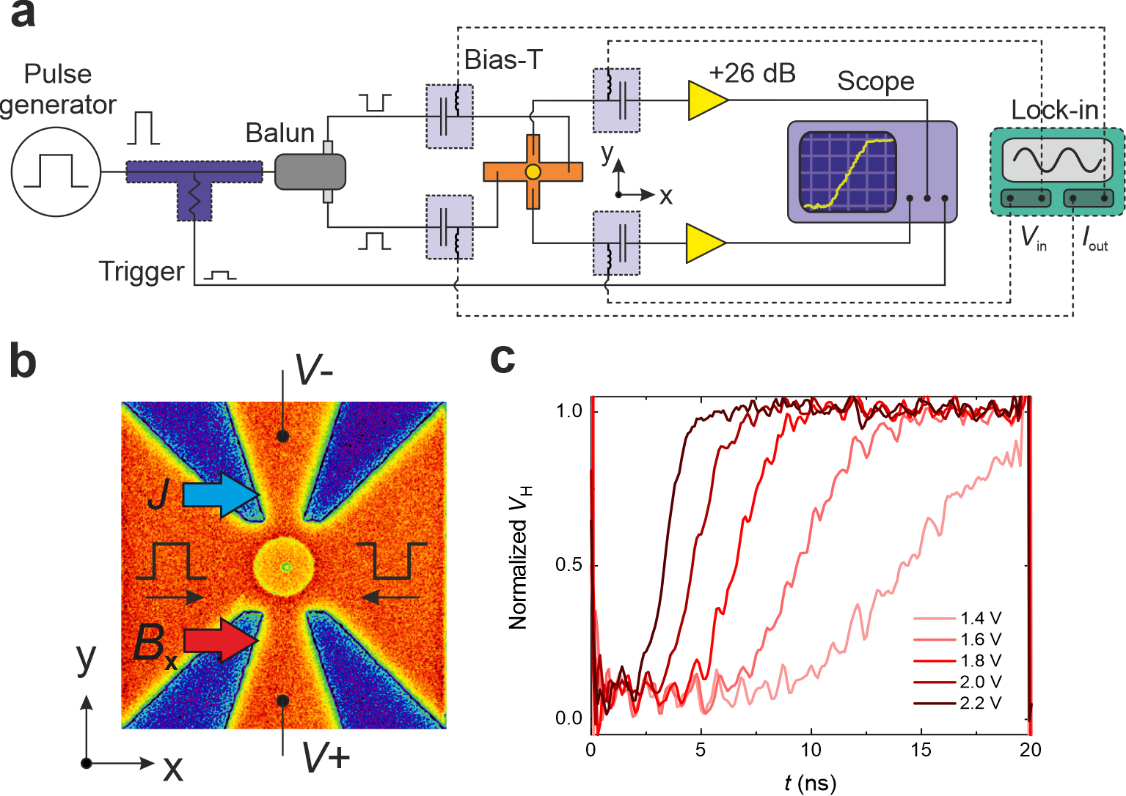
We have recently developed a new technique that solves this problem and allows for the detection of high-bandwidth Hall signals (Fig. 1). Our approach is conceptually simple, practical, and easily implementable. More importantly, it achieves the signal-to-noise ratio necessary to perform “single-shot” measurements, namely to detect individual transient events. This capability, which gives access to the stochastic components of the magnetotransport dynamics, is an important advantage over averaging techniques that rely on complex pump-probe setups or synchrotron radiation facilities. As any form transverse magnetoresistance equally fits our detection scheme, potential applications include time-resolved investigations of electrically- and thermally-generated spin currents and spin torques in magnetic materials, switching of antiferromagnets, as well as time-of-flight detection of skyrmion and domain walls in racetrack devices. Time-resolved Hall effect measurements can also probe the emergence of symmetry-breaking phase transitions in nonmagnetic electronic systems as well as current-induced transitions between quantum Hall and dissipative states in topological matter.
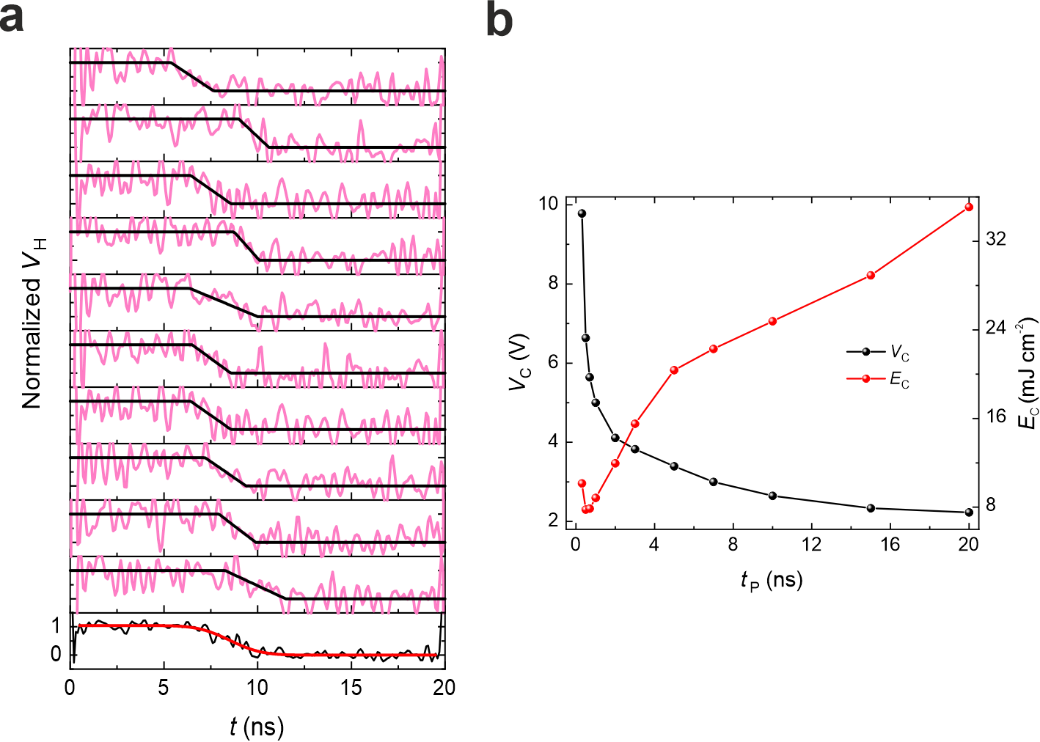
We have demonstrated the principles and efficacy of the technique by using the anomalous Hall effect to track the real-time switching of ferrimagnetic GdFeCo induced by spin-orbit torques (Fig. 2). These systems are receiving increasing attention thanks to their fast current-induced dynamics, as demonstrated by the motion of domain walls at speeds larger than 1 km/s. Such unprecedented velocity, along with the tunable magnetic properties of ferrimagnets, makes them competitive alternatives to ferromagnets in spintronic devices. Our measurements have confirmed these results but have also disclosed that the overall speed of the magnetization switching is limited by an initial activation time of thermal origin. In addition, our single-shot measurements have revealed that both the activation phase and the magnetization reversal are widely distributed in time. Luckily, there is a simple strategy to both mitigate the randomness of the dynamics and limit the duration of the initial quiescent phase, namely, using a more intense but shorter current excitation. This solution is also energetically efficient since it curbs the minimum energy required to obtain the magnetization switching.
Overall, our study provides a simple route to time-resolved Hall effect measurements as well as first evidence of stochastic switching behavior in ferrimagnetic systems. Identifying the existence of non-reproducible effects and understanding their origin is a prerequisite for the correct design and functionality of future ferrimagnetic devices.
Selected publication
- Time-dependent multistate switching of topological antiferromagnetic order in Mn3Sn, G.K. Krishnaswamy, G. Sala, B. Jacot, C.-H. Lambert, R. Schlitz, P. Nöel, P. Gambardella, Phys. Rev. Appl. 18, 024064 (2022). Downloadarticle (PDF, 2.4 MB)vertical_align_bottom, Downloadsupplementary information (PDF, 1.7 MB)vertical_align_bottom
- Real-time Hall-effect detection of current-induced magnetization dynamics in ferrimagnets, G. Sala, V. Krizakova, E. Grimaldi, C.-H. Lambert, T. Devolder and P. Gambardella, Nat. Comm. 12, 656 (2021). Downloadarticle (PDF, 921 KB)vertical_align_bottom