Research
Magnets possess both strength and memory. These two properties make them useful for a broad variety of applications, which involve action at a distance or retention of digital information. The continuous trend towards miniaturization and integration of devices, however, poses formidable challenges to develop materials that exhibit robust magnetization when downscaled to nanometer dimensions. One of the primary goals of our group is thus to realize stable nanoscale magnets, from multilayer structures down to the ultimate limit of single atoms, and tune these systems to exhibit specific magnetic properties. Systems with reduced magnetic volume offer the additional possibility to control the magnetization by means of local electric fields and currents rather than by external fields, with significant gains in terms of speed of operation and energy consumption. Finding new methods to reversibly switch the magnetization in electric devices is another central theme of our research, which we develop starting from the synthesis of magnetic heterostructures up to the level of prototype memory and logic systems.
Both our long-term research goals rely heavily on understanding the role of interfaces to promote or inhibit magnetic interactions. On a fundamental level, we are investigating how the magnetization of transition-metal and rare earth atoms evolves as a function of coordination and oxidation state in insulating as well as metallic environments. These studies feature single-atom and single-molecule magnets as well as arrays of patterned nanomagnets, which provide the basis to refine the synthesis of materials with interface-modulated properties, both electrical and magnetic. On the device level, we use thin film deposition techniques, such as molecular beam epitaxy and magnetron sputtering, to fabricate heterostructures that support ultrafast current-induced magnetization switching. We investigate the combination of pure and alloyed transition-metal films with heavy metal and topological insulator layers to promote charge-to-spin conversion due to the spin Hall effect and interface-driven spin-orbit torques. We also explore unusual magnetoresistive effects that arise from spin-dependent scattering in metal/oxide bilayers, which can be used to sense the magnetization direction of insulators by electrical means.
In pursuit of these goals, we work with polarized x-ray absorption spectroscopy (XAS, XMCD) as the main element-specific magnetic characterization technique, in combination with scanning tunneling microscopy (STM) for nanoscale characterization and local electron spectroscopy measurements. We have further developed all-electrical measurement methods based on ac and dc Hall resistance and magnetoresistance measurements to perform vector measurements of current-induced spin torques and magnetization switching in thin films and model devices. Current efforts focus on developing spatially- and time-resolved optical and scanning probe techniques to measure electrically-induced spin currents and magnetization dynamics with sub-nm and sub-ns resolution. More details are given below and in the submenu items on the left of this page.
Spintronics
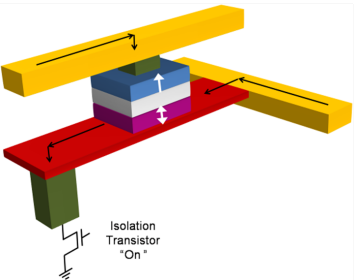
The spin of the electrons in magnetic as well as nonmagnetic materials can be used to represent and process information for sensing, memory, and logic applications. This area of research is called spintronics, in analogy with conventional electronics where the electric charge is used to store and transmit information. read more
Magnetism at the Nanoscale
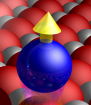
It is well known that the magnetic properties of materials depend on their chemical composition and crystal structure. When reducing the dimensions of a material system to the nanometer scale, however, other effects come into play. For example, magnetic nanoparticles tend to become "superparamagnetic", antiferromagnets become unstable, whereas nonmagnetic materials might become magnetic. As the surface-to-volume ratio increases in small structures, interfacial effects become dominant. Such effects can result in increased magnetic anisotropy, proximity magnetism, and novel types of magnetic coupling that can be exploited to control the magnetization of small systems. read more
Molecular Magnetism
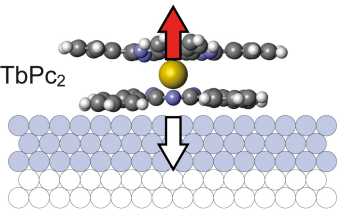
Magnetism in condensed matter is typically a collective phenomenon in which a multitude of spins interact together. Magnetic molecules, on the other hand, provide a unique oppportunity to study the behavior of individual spins as a function of the chemical environment. Some molecules, called single molecule magnets, even present hysteretic magnetic properties, similar to bulk magnets. The origin of magnetic hysteresis in molecular systems offers clues on the role of magnetic anisotropy as well as electronic, hyperfine, and vibrational quantum excitations in stabilizing or destabilizing the magnetic moment of small systems. Further, magnetic molecules can be deposited on inorganic ferromagnets and antiferromagnets in order to create hybrid metal-organic complexes, which have very different properties compared to other nanoscale systems. Read more