Unidirectional Magnetoresistance
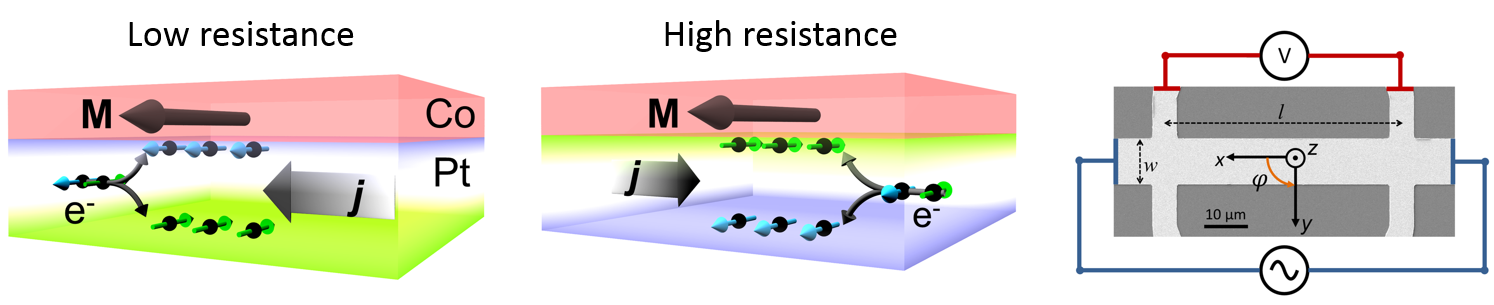
Magnetoresistance, the change of the electrical resistance of a material upon application of a magnetic field or rotation of the magnetization, is arguably the oldest manifestation of the interplay between charge transport and magnetism. Since William Thomson (later Lord Kelvin) discovered in 1856 that “the conducting power of an iron plate, when magnetized, became greater across than along the lines of magnetization”, magnetoresistance has become an invisible companion of our lives, notably in miniature sensors that measure linear position, angle, and rotation speed, as found in cars, as well as in electronic compasses that measure the Earth's magnetic field or the electric current in a conductor. Yet, more than 150 years later, magnetoresistance still holds hidden surprises, revealing fundamental aspects of electron conduction and enabling new technological applications. The most famous example concerns the discovery of the giant magnetoresistance effect (GMR) by Albert Fert and Peter Grünberg, which was awarded the 2007 Nobel Prize in Physics. This effect was discovered in multilayer structures alternating thin films of a ferromagnetic material, such as iron or cobalt, and a nonmagnetic spacer, such as copper, where the electrical resistance changes from low for parallel alignment of the magnetization in adjacent ferromagnetic layers to high for antiparallel alignment. GMR, together with its counterpart in tunnel junction heterostructures (TMR or tunneling magnetoresistance), is widely used today in magnetic field sensors and to read data in computer hard disk drives.
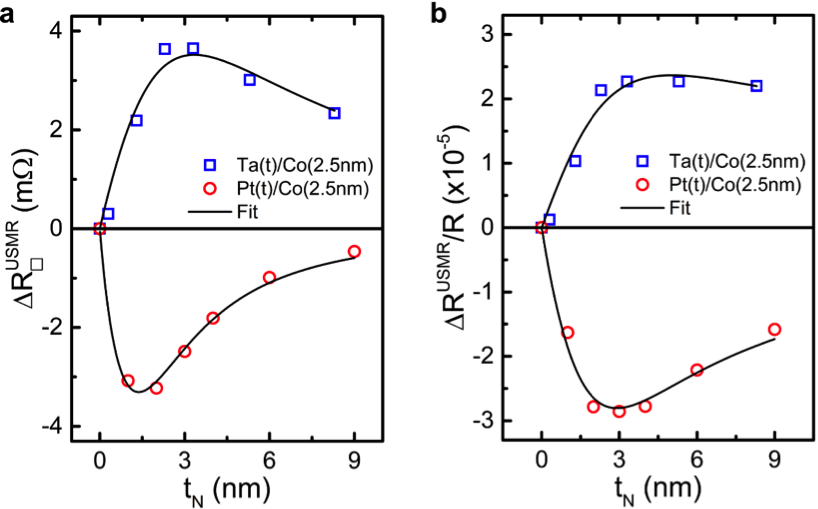
Due to the principle of microscopy irreversibility, however, which states that the way in which particles moves is symmetric with respect to the inversion of time, most magnetoresistance phenomena do not depend on the direction of electron flow, i.e., on the direction of the electric current. We have recently found an exception to this behavior, demonstrating a unidirectional magnetoresistance effect that depends on the sign of the current injected into a bilayer film composed of a nonmagnetic metal and a single ferromagnetic layer. This peculiar type of magnetoresistance is based on the “spin Hall effect”, namely the ability of certain nonmagnetic materials to deviate electrons with opposite spin magnetic moment in opposite directions. In such a material, typically a heavy metal such as tantalum or platinum, electrons having the same magnetization direction accumulate at each interface of the conductor. When a ferromagnet is placed on top of the heavy metal, the total electrical resistance is found to depend on the relative alignment of the current-induced electron spin accumulation and the magnetization of the ferromagnet. This is similar to GMR, but not identical. The main difference being that this so-called unidirectional spin Hall magnetoresistance is not only a property of the material, but also directly proportional to the amount of current injected into the system, since the current itself creates the spin accumulation at the interface between the nonmagnetic and magnetic conductors.
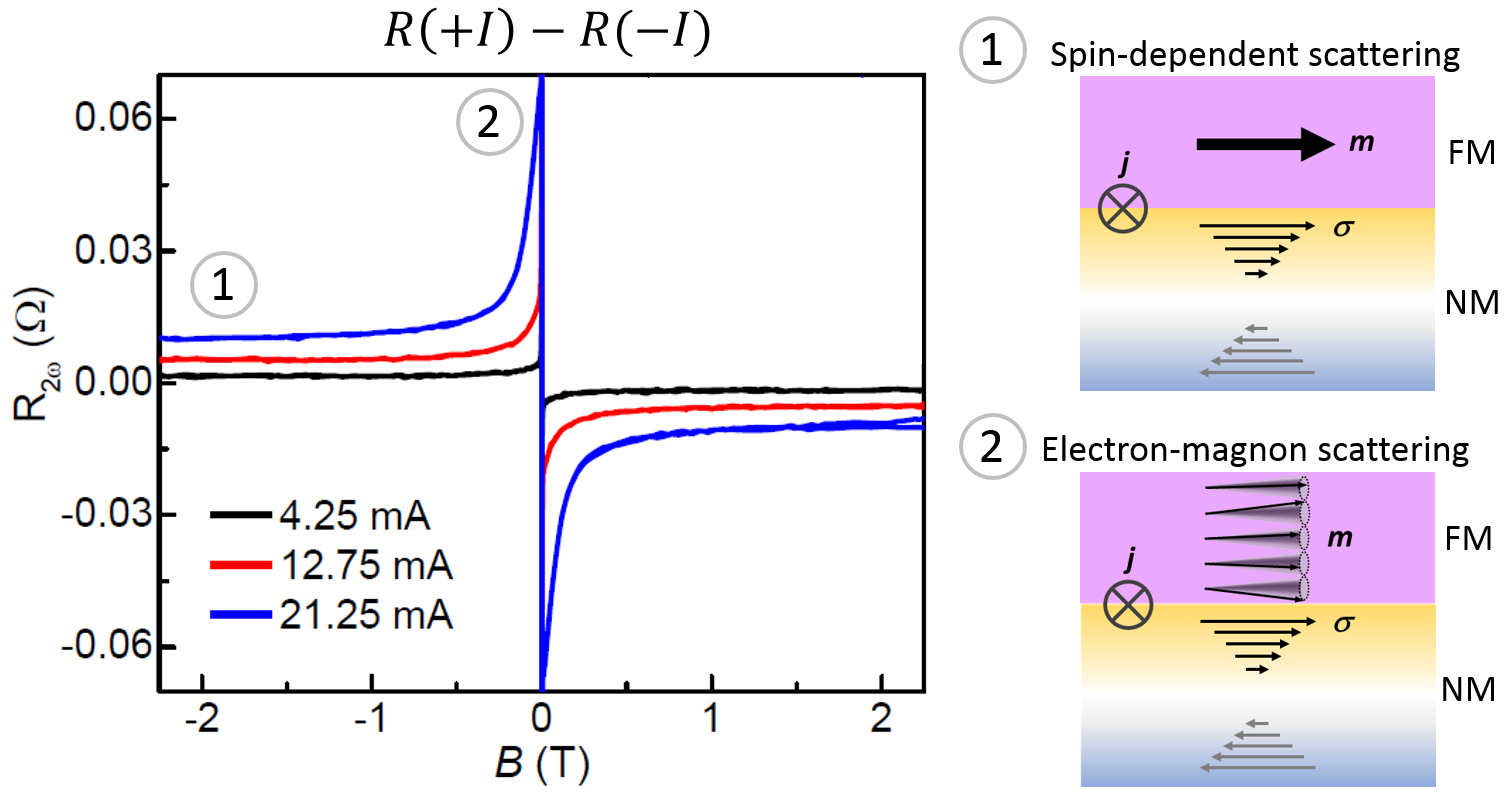
The existence of such a unidirectional magnetoresistive effect has both fundamental and practical implications. Identifying which symmetries survive the breakdown of the microscopic reversibility laws in the nonlinear regime is central to the understanding of electron transport phenomena, particularly in mesoscopic and magnetic conductors where such effects can have thermoelectric and magneto-optical counterparts. Moreover, although the discovered effect is very small relative to GMR, we are confident that certain classes of materials, including topological insulators and two-dimensional systems, will show enhanced unidirectional magnetoresistance properties. This may be useful to fabricate sensors and detect magnetization switching in two-terminal spintronic devices by a simple resistance measurement.
Unidirectional magnetoresistance in ferromagnetic microhelices
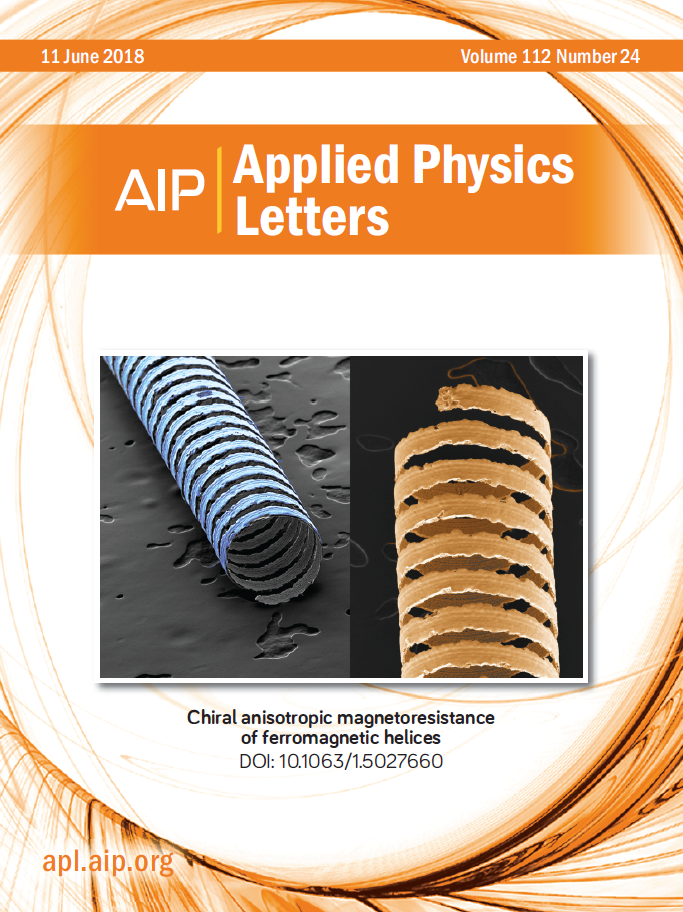
We investigate the anisotropic magnetoresistance (AMR) of ferromagnetic CoNi microhelices fabricated by electrodeposition and laser printing.
Conductors with twisted geometries also host exotic and nonlinear magnetoresistance effects. For example, helices with a three-dimensional winding display a characteristic angular and field-dependence of the anisotropic magnetoresistance (AMR) due to the competition between helical shape anisotropy and external magnetic field. Moreover, there is a unidirectional contribution to the AMR that scales proportionally to the applied current and depends on the helix chirality. This contribution originates from the solenoidal magnetic field induced by the current flowing in the helix, which modifies the orientation of the magnetization relative to the current and, hence, the magnetoresistance. These results underline the interest of three-dimensional curved geometries to tune the AMR and realize tubular magnetoresistive devices.
Selected publications
- Origins of the Unidirectional Spin Hall Magnetoresistance in Metallic Bilayers, C. O. Avci, J. Mendil, G. S. D. Beach, and P. Gambardella, Phys. Rev. Lett. 121, 087207 (2018). Download article (PDF, 322 KB)
- Unidirectional spin Hall magnetoresistance in ferromagnet/normal metal bilayers, C. O. Avci, K. Garello, A. Ghosh, M. Gabureac, S. F. Alvarado, and P. Gambardella, Nature Physics 11, 570 (2015). Download article (PDF, 666 KB)
- Magnetoresistance of heavy and light metal/ferromagnet bilayers, C. O. Avci, K. Garello, J. Mendil, A. Ghosh, N. Blasakis, M. Gabureac, M. Trassin, M. Fiebig, and P. Gambardella, Appl. Phys. Lett. 107, 192405 (2015). Download article (PDF, 1.1 MB)
- A multi-state memory device based on the unidirectional spin Hall magnetoresistance, C.O. Avci, M. Mann, A. J. Tan, P. Gambardella, and G. S. D. Beach, Appl. Phys. Lett. 110, 203506 (2017). Download article (PDF, 786 KB)
- Chiral anisotropic magnetoresistance of ferromagnetic helices, H. Maurenbrecher, J. Mendil, G. Chatzipirpiridis, M. Mattmann, S. Pané, B. J. Nelson, and P. Gambardella, Appl. Phys. Lett. 112, 242401 (2018). Download article (PDF, 264 KB)