Electron Paramagnetic Resonance Spectroscopy by STM
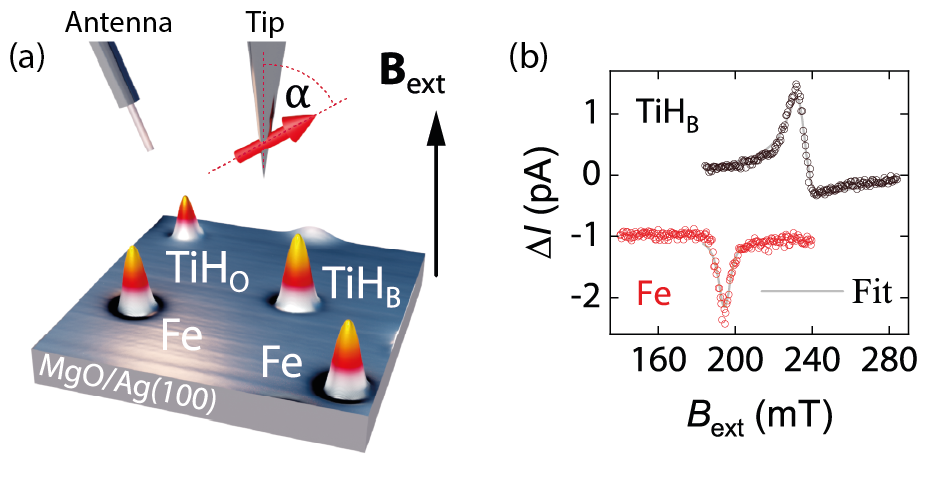
Magnetic resonance techniques are widely employed for probing the electronic and magnetic properties of solids, liquids, and molecules as well as for their elemental and structural characterization. These techniques probe with high precision the excitation of the magnetic states of an atom, or of a nucleus, and provide information on their chemical environment. For instance, electron paramagnetic resonance (EPR) is routinely used for non-invasive spin detection in materials science and chemistry research [1], e.g. for the identification of free radicals in chemical reactions, detection of spin-labeled molecules in biological systems, and the study of molecular nanomagnets. However, conventional magnetic resonance techniques can only detect a macroscopic number of spins (~107 electron spins, ~1012 nuclear spins) and have poor spatial resolution ranging from 20 µm to 1 mm with sample volumes of a couple of microliters.
A great leap towards single spin detection was made with the demonstration of EPR spectroscopy using the tip of a scanning tunneling microscope (STM). After earlier attempts, Baumann et al. [2] were the first to convincingly demonstrate EPR of single atoms on a surface. This approach uses an electrical method both to drive and read the spin, circumventing the photon quenching of optical methods. In its original conception, EPR-STM realizes a resonance experiment on a single spin on a surface that, combined with the spatial accuracy of STM, permits sub-atomic resolution magnetic resonance imaging, see Figure. In EPR-STM experiments, an external magnetic field splits the energy of the states of the magnetic atom under investigation. A resonant microwave voltage fed to the STM tunnel junction, either directly through the tip or via an antenna, induces transitions between these Zeeman-split states. Upon resonance, the spin-dependent conductivity of the tunnel junction varies, which is sensed by a spin-polarized STM tip through a magneto-resistive effect. EPR-STM outperforms conventional magnetic resonance techniques by seven orders of magnitude in sensitivity, which allows for probing the magnetism of individual atoms [2] including their nuclear spins [3] and even makes it possible to carry out magnetic resonance imaging experiments. Moreover, pulsed rf schemes have been used to coherently drive EPR excitations in single atoms making use of Ramsey and Hahn echoes techniques [4]. These promising developments open the way for further applications of EPR-STM, such as the storage and retrieval of quantum information from surface spins, measurements of the relaxation time of single molecule magnets, and the characterization of active sites and intermediate reaction species in catalysis.
In our laboratory, we have recently implemented EPR-STM using a novel antenna coupling scheme that provides high excitation power levels, as required for coherent manipulation of individual spins [5]. Using our large range of rf excitation power levels, we carried out an extensive experimental study and modeling of EPR-STM of Fe and hydrogenated Ti atoms on a MgO surface, shedding light on the mechanism driving the EPR excitations [6]. In our research we seek to broaden the applicability of EPR-STM to new systems and we donstrated that EPR-STM can be used to identify the charge state of alkali metal atoms and dimers on a ultrathin MgO film exhibiting a distinct behavior from adsorption on the bulk oxide [7].
References
[1] M.M. Roessler and E. Salvadori, Principles and applications of EPR spectroscopy in the chemical sciences, Chemical Society Reviews, 47, 2534-2553 (2018).
[2] S. Baumann, W. Paul, T. Choi, C.P. Lutz, A. Ardavan, and A.J. Heinrich, Electron paramagnetic resonance of individual atoms on a surface, Science, 350, 417 (2015).
[3] P. Willke, Y. Bae, K. Yang, J.L. Lado, A. Ferron, T. Choi, A. Ardavan, J. Fernandez-Rossier, A.J. Heinrich, and C. P. Lutz, Hyperfine interaction of individual atoms on a surface, Science, 362, 336-339 (2018).
[4] K. Yang, W. Paul, S.H. Phark, P. Willke, Y. Bae, T. Choi, T. Esat, A. Ardavan, A.J. Heinrich, and C.P. Lutz, Coherent spin manipulation of individual atoms on a surface, Science, 366, 509-512 (2019).
[5] T.S. Seifert, S. Kovarik, C. Nistor, L. Persichetti, S. Stepanow, and P. Gambardella, Single-atom electron paramagnetic resonance in a scanning tunneling microscope driven by a radio-frequency antenna at 4 K, Physical Review Research, 2, 013032 (2020).
[6] T.S. Seifert, S. Kovarik, D.M. Juraschek, N.A. Spaldin, P. Gambardella, and S. Stepanow, Longitudinal and transverse electron paramagnetic resonance in a scanning tunneling microscope, Science Advances, 6, eabc5511 (2020).
[7] S. Kovarik, R. Robles, R. Schlitz, T. S. Seifert, N. Lorente, P. Gambardella, S. Stepanow, Electron paramagnetic resonance of alkali metal atoms and dimers on ultrathin MgO, Nano Letters, 22, 4176-4181 (2022).
Reference
[1] M.M. Roessler and E. Salvadori, Principles and applications of EPR spectroscopy in the chemical sciences, Chemical Society Reviews, 47, 2534-2553 (2018).
[2] S. Baumann, W. Paul, T. Choi, C.P. Lutz, A. Ardavan, and A.J. Heinrich, Electron paramagnetic resonance of individual atoms on a surface, Science, 350, 417 (2015).
[3] P. Willke, Y. Bae, K. Yang, J.L. Lado, A. Ferron, T. Choi, A. Ardavan, J. Fernandez-Rossier, A.J. Heinrich, and C. P. Lutz, Hyperfine interaction of individual atoms on a surface, Science, 362, 336-339 (2018).
[4] K. Yang, W. Paul, S.H. Phark, P. Willke, Y. Bae, T. Choi, T. Esat, A. Ardavan, A.J. Heinrich, and C.P. Lutz, Coherent spin manipulation of individual atoms on a surface, Science, 366, 509-512 (2019).
[5] T.S. Seifert, S. Kovarik, C. Nistor, L. Persichetti, S. Stepanow, and P. Gambardella, Single-atom electron paramagnetic resonance in a scanning tunneling microscope driven by a radio-frequency antenna at 4 K, Physical Review Research 2, 013032 (2020). Download article (PDF, 1.8 MB)
[6] T.S. Seifert, S. Kovarik, D.M. Juraschek, N.A. Spaldin, P. Gambardella, and S. Stepanow, Longitudinal and transverse electron paramagnetic resonance in a scanning tunneling microscope, Science Advances, 6, eabc5511 (2020). Download article (PDF, 7.3 MB)